Hertz was a careful investigator, cautious of the sweeping claims being made by Poynting, Lodge, and Heaviside for how electromagnetism works. While he made ample use of fields or “lines of force” in his own investigations, Hertz preferred to think of them as “states” imposed on matter or space, not as physically real entities. “For the conception employed by Faraday, and developed by Poynting, of a motion of the lines of force relatively to the surrounding medium, is indeed a highly remarkable one, and may be capable of being worked out; but it is entirely different from the view here followed, according to which the lines of force simply represent a symbol for special conditions of matter” [[i]].
Hertz’s prudent skepticism did not prevent him from applying conceptions like fields and energy flow to the end of understanding electromagnetic systems and their behavior, however. In particular, Hertz offered a remarkably intuitive picture of energy flow around oscillating dipoles. Energy oscillates in and out near a dipole, yet with each oscillation, a little more energy travels out than flows back in.
While the oscillator is at work the energy oscillates in and out through the spherical surfaces surrounding the origin. But the energy which goes out during each period of oscillation is greater than that which returns, and is greater by the same amount for all the surfaces. This excess represents the loss of energy due to radiation during each period of oscillation [[ii]].
At a radial distance equal to 7% of a wavelength (r = 0.07 λ), three quarters of the energy pushed out falls back in. At 10% of the wavelength, it’s fifty-fifty. By a radial distance of 30% of a wavelength, virtually all the energy is flowing out. Figure 4.54 illustrates the magnitude of these energy flow in (Uin), energy flow out (Uout) and the radiation energy (Urad) around a dipole [[iii]]. We will examine this behavior in more detail in Chapter 8.
Hertz was troubled by the complexities of following energy flow. If a drive pulley connects to a follower pulley by a strap, applying a torque to the drive pulley makes the follower pulley rotate the same way. The strap moves toward the drive pulley, while the energy flows in the opposite direction.
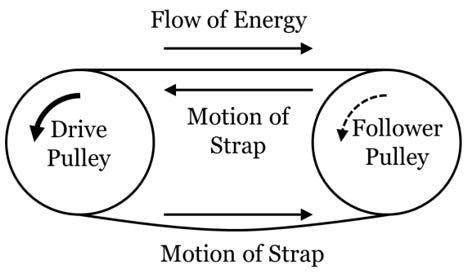
Figure 4.55 shows this pulley configuration. The forward motion of the strap exerts the force; the energy flows in reverse as the first wheel drives the second. Remember the example of the pulley and the strap. We will soon appreciate that electromagnetics works similarly. Fields move one way. Energy moves another way. Hertz noted, “[t]here are difficulties here which badly need clearing up” [[iv]]. Chapter 8 addresses how radiation works in much more detail.
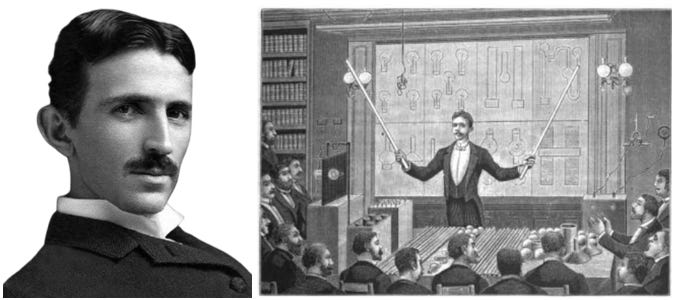
In 1892, Nikola Tesla (1856–1943) travelled from the U.S. to Europe for a well-received lecture tour [[vii]]. Appearing before the London Institute of Electrical Engineers, Tesla gave a demonstration-packed lecture that brought out even the normally reclusive Oliver Heaviside [[viii]]. An encore performance the next evening packed the Royal Institution as well. Figure 4.56 shows Tesla and an engraving of him presenting the same lecture in France a couple weeks later. Fresh from his design and implementation of the alternating current electrical distribution system we still employ today, Tesla promised further remarkable innovations in the area of high voltage and high frequency engineering.
Ultimately, the U.S. Supreme Court upheld Tesla as the inventor of radio [[ix]]. This pyrrhic victory came months after the inventor’s death in 1943, and may have been influenced by the fact that Tesla’s late rival, Italian Guglielmo Marconi (1874–1937), was a national of a hostile power. Despite the remarkable success of Tesla’s earlier work, his later work remains poorly understood and outside the mainstream.
Electromagnetism obeys what’s called the “superposition principle:” the whole is the sum of its parts. Each of the parts may be treated in isolation, because – under most conditions – one part does not affect or influence the others. Hertz was slow to see the practical implications of his discovery in light of the superposition principle. Others had more foresight.
On the eve of Tesla’s 1892 lecture, British physicist William Crookes (1832–1919) rushed to get his remarkable predictions of our wireless future into print. Crookes foresaw how Hertzian physics would enable wireless communication in light of the principle of superposition:
Rays of light will not pierce through a wall, nor, as we know only too well, through a London fog. But the electrical vibrations of a yard or more in wave-length of which I have spoken will easily pierce such mediums, which to them will be transparent. Here then, is revealed the bewildering possibility of telegraphy without wires, posts, cables, or any of our present costly appliances. Granted a few reasonable postulates, the whole thing comes well within the realms of possible fulfillment [[x]].
The practical implication of the superposition principle was that–given suitable electronics to isolate the individual frequency components–any number of channels were available merely by using a different frequency, or equivalently, a different wavelength. As Crookes further speculated:
Any two friends living within the radius of sensibility of their receiving instruments, having first decided on their special wavelength and attuned their respective instruments to mutual receptivity, could thus communicate as long and as often as they pleased by timing the impulses to produce long and short intervals in the ordinary Morse code [[xi]].
In 1894, Oliver Lodge (1851–1940) gave his own lecture before the Royal Institution on the work of Hertz and some of his successors [[xii]]. Lodge demonstrated a coherer–one of the first practical radio receivers. In a few short years, Lodge not only devised the first practical tuned radio architecture, but also invented some of the first practical antenna designs [[xiii]]. However, a young Italian inventor, Guglielmo Marconi (1874–1937), was most responsible for making radio a commercial success [[xiv]]. We’ll see more of the fruits of his labors in Chapter 6. The scope of this gilded-age radio science was truly remarkable, and anticipated work brough to fruition decades or even a century later.
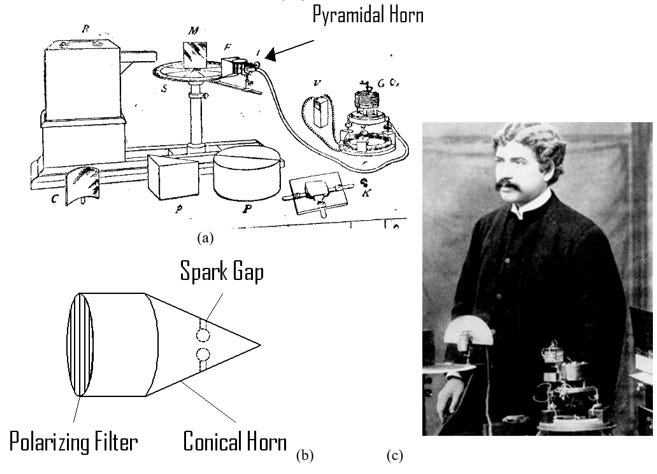
Jagadis (alternatively, Jagadish) Chandra Bose (1858–1937) invented horn antennas while pioneering millimeter-wave wireless in the 1890s [see Figure 4.57(c)]. In a public demonstration in 1895, Bose used electromagnetic waves to ring a bell remotely and to detonate gunpowder. In 1897, Lord Rayleigh (1842–1919) invited Bose to London to present his research to the Royal Society in London. Bose demonstrated a complete system for transmitting and receiving radio waves at millimeter-wave frequencies of around 60 GHz. This system included what Bose dubbed “collecting funnels,” the first horn antennas. Bose used a spark-gap transmitter and a semiconductor junction as a receiver. Figure 4.57(a) reproduces one of Bose’s figures showing a pyramidal horn. Figure 4.57(b) shows a sketch of one of Bose’s conical horn antennas. Additional details of nineteenth-century microwave and millimeter-wave research are available elsewhere [[xv], [xvi]]. Bose’s pioneering work would be largely forgotten for several decades before being rediscovered.
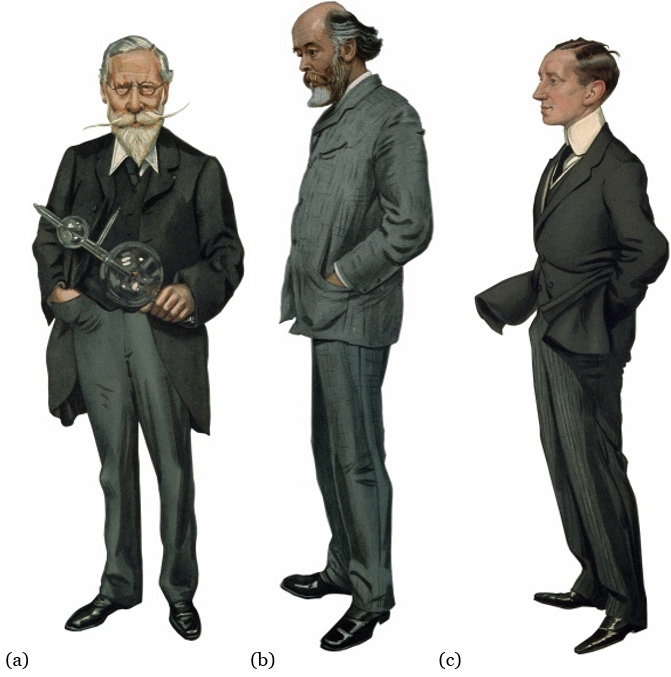
The wireless revolution was made possible by Hertz’s remarkable discoveries. The practical implications remain obvious today. The physical implications of Hertz’s work, however, are often forgotten. While pioneering radio scientists like Crookes, Lodge and Marconi, shown in Figure 4.58, explored the practical aspects of radio science, Hertz understood that there were deeper implications for his discoveries. Hertz caught electromagnetic energy in the act of moving from place to place. His results validated the conception of Faraday and Maxwell that electricity and magnetism involve processes taking place in the space between the source and the detector. As Hertz explained:
In the sense of our theory we more correctly represent the phenomena by saying that fundamentally the waves which are being developed do not owe their formation solely to processes at the origin, but arise out of the conditions of the whole surrounding space, which latter, according to our theory, is the true seat of the energy [[xvii]].
With Hertz’s discovery of radio waves (1888‑1889) the triumph of Faraday’s field point of view appeared complete [[xviii]]. Hertz noted:
The preponderance of such [action-at-a-distance] forces in theory has long been sanctioned by science, but has always been accepted with reluctance by common sense; in the domain of electricity these forces now appeared to be dethroned from their position by simple and striking experiments [[xix]].
As Hertz labored to make sense of Maxwell’s theory, he found himself drawn to the same simplifications and interpretations of Maxwell as found by Heaviside.
….I have been led to endeavor for some time past to sift Maxwell’s formulae and to separate their essential significance from the particular form in which they first happened to appear. The results at which I have arrived are set forth in the present paper. Mr. Oliver Heaviside has been working in the same direction ever since 1885. From Maxwell’s equations he removes the same symbols [the potentials] as myself; and the simplest form which these equations thereby attain is essentially the same as that which I arrive. In this respect, then, Mr. Heaviside has the priority…” [[xx]].
Cautious of reasoning beyond the direct implications of his results, however, Hertz acknowledged:
To the question, ‘What is Maxwell’s theory?’ I know of no shorter or more definitive answer than the following:–Maxwell’s theory is Maxwell’s system of equations [[xxi]].
Others were guided by different premises, and their ideas would shape the interpretation of the discoveries to come.
Next time: 4.6.2 The Accelerating Charge Model: The Radiation of One Charge Accelerating
Enjoy the article, but maybe not quite enough to subscribe?
Then click on the button to buy me a coffee. Thanks!
Full Table of Contents [click here]
Chapter 4 Electromagnetism Comes of Age
4.5 An Introduction to Electromagnetic Models
4.5.3 A Synthesis
4.6 Hertz & Radiation Fields
4.7 How Does Radiation Work?
4.8 Summary & Conclusions
Follow Online:
You may follow me online in other places as well:
Telegram: 𝔸𝕖𝕥𝕙𝕖𝕣𝕔𝕫𝕒𝕣'𝕤 𝔸𝕖𝕥𝕙𝕖𝕣𝕤𝕥𝕣𝕖𝕒𝕞
Gab: @aetherczar
Twitter: @aetherczar
Amazon: Hans G. Schantz
References
[[i]] Hertz, H., Electric Waves, London: MacMillan and Co., 1893, p. 255.
[[ii]] Hertz, H., Electric Waves, London: MacMillan and Co., 1893, p. 149.
[[iii]] Schantz, Hans G., The Art and Science of Ultrawideband Antennas, 2nd ed., Boston: Artech House, 2015, p. 349.
[[iv]] Hertz, H., Electric Waves, London: MacMillan and Co., 1893, p. 277.
[[v]] Tesla, aged 34, circa 1890. Photo by Napoleon Sarony. See: https://en.wikipedia.org/wiki/Nikola_Tesla#/media/File:Tesla_circa_1890.jpeg
[[vi]] Anonymous, “Mr. Tesla’s Experiments on Alternating Currents of Great Frequency,” Scientific American, March 26, 1892, p. 195.
[[vii]] Anonymous, “Mr. Tesla Before the London Institution of Electrical Engineers – and the Royal Institution,” The Electrical Engineer, February 10, 1892, p. 139.
[[viii]] Seifer, Marc J., Wizard: The Life and Times of Nikolas Tesla, Citadel Press, 2011. Listened to the audiobook, so I don’t have a page number. I confirmed this claim via email with the author.
[[ix]] Tesla, Nikola, “System of transmission of electrical energy,” U.S. Patent 645,576, March 20, 1900.
[[x]] Crookes, William, “Some Possibilities of Electricity,” Fortnightly Review, February 1, 1892, pp. 174–176. This article is available online at: earlyradiohistory.us/1892fort.htm at the time of this writing.
[[xi]] Crookes, William, “Some Possibilities of Electricity,” Fortnightly Review, February 1, 1892, pp. 174–176. This article is available online at: earlyradiohistory.us/1892fort.htm at the time of this writing.
[[xii]] Lodge, Oliver, The Work of Hertz and Some of His Successors: Being the Substance of a Lecture Delivered at the Royal Institution on Friday Evening June 1, 1894 with Additions and Appendices, London: The Electrician, 1894.
[[xiii]] Lodge, Oliver, “Electric telegraphy,” U.S. Patent 609,154, August 16, 1898.
[[xiv]] Garratt, G.R.M., The Early History of Radio from Faraday to Marconi, London: IEE, 1994, p. 69.
[[xv]] Emerson, Darrel T., “The work of Jagadis Chandra Bose: 100 years of millimeter wave research,” IEEE Transactions on Microwave Theory and Techniques, Vol. 45, No. 12, December 1997, pp. 2267‑2273. See also www.tuc.nrao.edu/~demerson/bose/bose.html. The author is indebted to Dr. Emerson for his insightful presentation and for permission to reproduce Figures 2.5(a) and 2.5(c).
[[xvi]] Ramsay, John F., “Microwave Antenna and Waveguide Techniques Before 1900,” Proceedings of the IRE, Vol. 46, No. 2, February 1958, pp. 405–415.
[[xvii]] Hertz, H., Electric Waves, London: MacMillan and Co., 1893, p. 146.
[[xviii]] Hertz, Heinrich, Electric Waves, London: MacMillan and Co., 1893. See the insightful preface by Lord Kelvin for the philosophical implications of Hertz’s discoveries.
[[xix]] Heinrich Hertz, Electric Waves: Being Researches on the Propagation of Electric Action with Finite Velocity Through Space, (D.E. Jones, Trans.), (New York: Macmillan and Co., 1893), p. 18.
[[xx]] Hertz, H., Electric Waves, London: MacMillan and Co., 1893, pp. 196-197.
[[xxi]] Hertz, Electric Waves, pp. vi-vii.
"The scope of this gilded-age radio science was truly remarkable, and anticipated work brought to fruition decades or even a century later."
When I worked for Lucent Technologies over 20 years ago, I was privileged to work with some real researchers in fundamental materials science for communications applications, and I got to see the first design for a mobile telephony system. IIRC it was designed in 1944.
Did anyone else get two copies of this in their inbox? Trying to figure out if it's something I did.