4.7 Summary & Conclusions
Models - An Essential Part of Understanding Electromagnetism, And Everything Else
Some mail servers may truncate this post. Click on the headline to view it online.
“Men of acute intelligence,” William Gilbert (1544-1603) avowed, “without actual knowledge of facts, and in the absence of experiment, easily slip and err” [[i]]. Even careful observers may be mistaken. Gilbert, himself, missed identifying electric repulsion, saying: “Electrics attract objects of every kind ; they never repel” [[ii]].
The ingenious theory of James Clerk Maxwell (1831–1879) did not achieve immediate acceptance, and even those most ready to entertain his ideas were pessimistic. A small band of Maxwellian enthusiasts pursued Maxwell’s tentative beginning and explored its logical consequences. Oliver Heaviside (1850–1925) labored to make sense of Maxwell. Failing in his attempt, Heaviside adapted Clerk Maxwell’s equations to vector calculus and rewrote Maxwell’s theory in its modern form. Between them, John Henry Poynting (1852–1914) and Heaviside developed the theory of electromagnetic energy flow, the theory that describes how electromagnetic energy moves from place to place. Finally, the physics that had so troubled Faraday of how charges and currents over here could interact with charges and currents over there began to be understood in detail.
The Poynting-Heaviside theory – that fields carried and guided the flow of energy – defied long-established, conventional thinking of electricity as a fluid. English investigators in particular were fond of making models to explain and illustrate electromagnetic behavior. Today, we employ their most successful models, like the field theory and the fluid theory, to understand electromagnetism, and electronics, respectively, but there are many other models that helped explain certain phenomena yet were cast aside in favor of more sophisticated models in light of growing experimental evidence and understanding.
From the earliest awareness of electric and magnetic phenomena, investigators tried to make sense of it. These phenomena involved “souls,” in the earliest views, because, like living things, electricity and magnetism gave rise to motion and movement. In a view that anticipated field theory, William Gilbert (1544–1603) proposed that magnetism involved an invisible orb or zone of “virtue” within which magnetic effects could arise. The 1629 diagram of field lines by Niccolò Cabeo (1586–1650) would have been a credit to Faraday, himself, but this suggestive depiction was ahead of its time. Electricity was some kind of atmosphere or effluvia, in later views, because it seemed to be most effective when an object was mechanically excited, as in the electrical machine of Otto von Guericke (1602–1686).
The extensive program of experimentations undertaken by Stephen Gray (1666–1736) enabled John Theophilus Desagulier (1683–1744) to identify the concept of conductors and insulators–the seeds of what would become the fluid theory of electricity. The French Court became a center for electrical research and demonstrations with Charles François de Cisternay du Fay (1698–1739) and the Abbé Jean-Antoine Nollet (1700–1770) devising a “two-fluid” theory to explain the phenomena. The Leyden Jar pioneered by Pieter van Musschenbroek (1692–1761) allowed electricity to be stored and concentrated. Scientific research was “a cultured pastime of the genteel educated elite, performed in drawing rooms and salons, with women taking part [[iii]].”
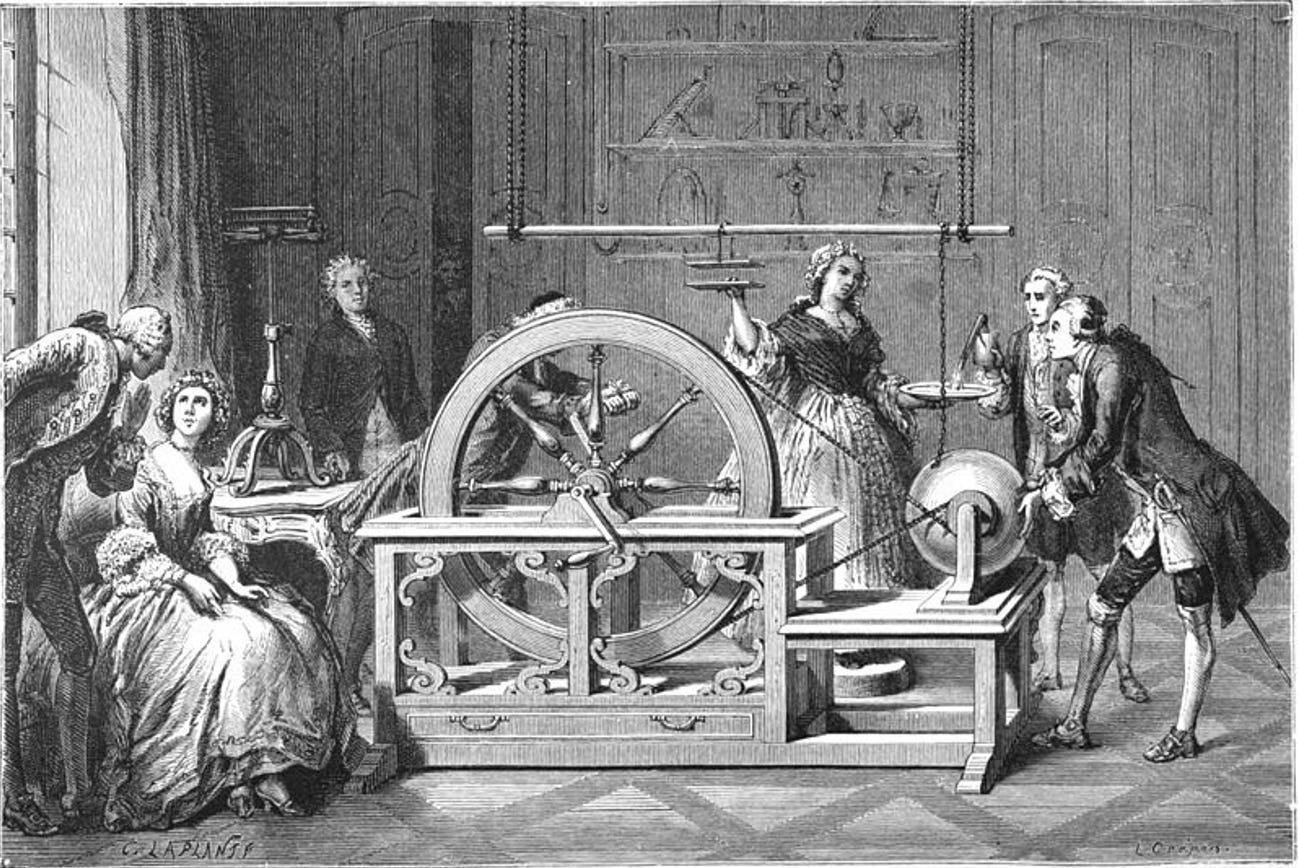
The science of electricity was ripe for a more rigorous systematization and application to practical uses. Joseph Henry (1797–1878), generously observed:
NO PART of the writings of Franklin exhibits his sagacity and his power of scientific generalization in a more conspicuous light than his theory of electricity. The talent to discover isolated facts in any branch of science, although possessed by a few, is comparatively inferior to that characteristic of mind which leads to the invention of an hypothesis embracing in a few simple propositions whole classes of complete phenomena [[v]].
Benjamin Franklin (1705–1790) and William Watson (1715–1787) between them laid out a one fluid theory of electricity that – with the identification of negatively charged electrons and their flow as the origin of “charge” and “current,” respectively – still holds up today. An excess of electricity (a deficit of electrons) leads to a positive charge. A deficit of electricity (a surplus of electrons) is a negative charge. The flow of electricity from one place to another is a current. Franklin recognized that his electrical fluid acted at a distance: through the glass of a Leyden jar or electrostatically attracting objects at a short distance.
Joseph Priestley (1733–1804), John Robison (1739–1805), and Henry Cavendish (1731–1810) made remarkable advances in understanding electricity and the force exerted by charges. The contributions of the reclusive Cavendish in particular, awaited appreciation decades after his death.
The pioneering work of the Italian scientists, Luigi Aloisio Galvani (1737–1798) and Alessandro Volta (1745–1827), led to the invention of the battery, whose sustained and controlled electrical currents made possible further electromagnetic advances.
In 1831, Michael Faraday (1791–1867) discovered the principle of induction, that changing magnetic fields generate electricity, kicking off the study and application of alternating current (AC) circuits [[vi]]. The fluid theory of electricity could not entirely explain the behavior of AC circuits. The phenomena of skin depth, cross-coupling between nearby wires, and near-field induction challenged the simple depiction of the fluid theory.
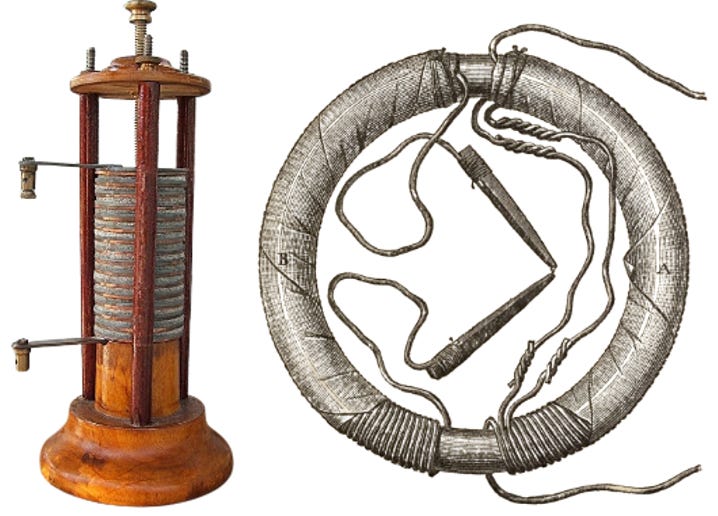
Faraday’s American contemporary, Professor Joseph Henry (1797–1878), demonstrated electric and magnetic induction at modest distances, a phenomenon that Lucius J. Phelps (1850–1925) and Thomas Edison (1847–1931) would exploit in their railway telegraphy inventions. William Preece (1834–1913) pushed magnetic science to its limits transmitting signals inductively across distances of a couple miles.
The technical fruits of these groundbreaking investigations are with us today in such applications as Radio-Frequency Identification (RFID), Near-Field Communications (NFC), and Near-Field Electromagnetic Ranging (NFER).
Charges and currents give rise to fields. Fields act upon charges and currents exerting the forces to make them move. The Poynting-Heaviside theory provides us with the missing link to understand the tripartite nature of electromagnetism. Fields guide energy, and the energy does the actual work on the charges.
The discoveries of Heinrich Hertz provided the missing link to make this all clear. Hertz discovered that the motions of charges and currents were not required to convey electromagnetic energy. Once the charges and currents launch an electromagnetic wave, the fields of the electromagnetic wave guide the flow of energy through space independent of any source charges.
While Hertz opined that “Maxwell’s theory is Maxwell’s system of equations,” clearly Hertz saw beyond the math to achieve a deeper understanding of what the math really meant. Writing in Great Men of Science, Nobel Laureate Philipp Lenard (1862–1947) confirmed:
It is very noteworthy that in the fifteen years between Maxwell’s publication of his work On Electricity and Magnetism and Hertz’s discoveries, a great deal had been written about ‘Maxwell’s theory’ and in particular concerning the electro-magnetic theory of light, and these subjects had been lectured upon at universities, yet not even the beginning of a way to the goal had been made clear, for people simply played about with Maxwell’s equations, but not with the ideas of Maxwell or Faraday; it was a mathematical game and not scientific research that was pursued, and the results were sterile.
Hertz was the first who not only understood the equations, and knew how to deal with them mathematically when necessary, but also saw the structure of ideas upon which they were based by their originator, and understood how to move about in it.
The equations are, so to speak, merely ground plans of this structure, and are far from being actual inhabitable apartments; the latter can only be produced by the architect, who knows how to grasp the ideas which have been put into the ground plan [[ix]].
With meticulous experiments and insightful theory, Hertz explained electromagnetic radiation. His results confirmed Faraday’s speculations, Maxwell’s theory, and the energy flow models of Poynting and Heaviside. Hertz’s discoveries opened up the world of wireless technology. As Hendrik Antoon Lorentz (1853–1928) observed:
It might even be questioned whether in electromagnetic phenomena the transfer of energy really takes place in the way indicated by Poynting's law… It must be observed however that there is no longer room for any doubt so soon as we admit that the phenomena going on in some part of the ether are entirely determined by the electric and magnetic force existing in that part. No one will deny that there is a flow of energy in a beam of light; therefore, if all depends on the electric and magnetic force, there must also be one near the surface of a wire carrying a current, because here, as well as in the beam of light, the two forces exist at the same time and are perpendicular to each other [[x]].
Emerging evidence that the transmission of electromagnetic effects is causal, progressive, and propagates at the speed of light eroded confidence in action-at-a-distance models. However, such models, like Weber’s, describe interactions between charges independent of any appeal to fields as an intermediary. Comparing and contrasting these models against Maxwellian approaches may yet yield novel insights.
“All models are wrong,” declared statistician George E. P. Box (1919–2013). “Some are useful” [[xi]]. Contrast that perspective with that of the “shut up and calculate” crowd who implicitly rely on physical models (like a single charge accelerating) without ever questioning their fundamental premises or seriously examining the implicit models they take for granted.
Consider what German physicist and science communicator, Sabine Hossenfelder (1976– ), has to say about trying to understand modern physics through models.
If you want to understand modern physics -- or really any abstract ideas -- you have to take it for what it is and stop trying to understand it through something else like it. There isn't anything else like it.
This is the problem with well-intended analogies like the rubber sheet for gravity or pairs of shoes for entangled particles or a spinning ball for spin or the like. They're all wrong and if you take them seriously they will just confuse you [[xii]].
If Faraday had taken this advice, electromagnetism might be vastly different today. Because Faraday didn't understand prevailing action-at-a-distance theories, he invented field theory. Trying to understand is the prerequisite of any fundamental discovery. Models are the means to that understanding. We can’t help but think in terms of models. Our only choice in the matter is whether those models are well-thought-out, or whether – eschewing models – we implicitly employ poorly-thought-out models, like the “radiation of one charge accelerating” model.
The Maxwellians offered us better advice. As George Francis FitzGerald (1851–1901) observed:
Before proceeding to explain how the electric and magnetic conditions of the ether may be illustrated by a model, it may be well to say a few words upon the general question of physical analogues. There is some danger in using physical analogues as assistants to our reasoning. There is no doubt but that a concrete mechanism that we can distinctly picture the working of is enormously easier to reason about than one of whose structure we know nothing, but only know general laws of its action. The danger is that we may be satisfied with an analogy, and mistake it for a likeness [[xiii]].
Wield them wisely and judiciously, and well-thought-out models are the key to unlock physical understanding. Wield them wantonly, assume they are what they model, and you fall into error. Refuse to wield them, and you surrender without a fight.
“Brother Potamian,” Michael Francis O’Reilly (1847–1917), and James J. Walsh (1865–1942), authors of Makers of Electricity (1909), offered this wisdom on the role on models in cognition:
History shows that scientific theories have their periods of infancy, maturity and decay. When they have served their purpose, like the scaffolding of a building, they are removed from sight and stored away, say, in a limbo of discarded philosophy, for use of the historian of science or of the metaphysician writing on the nature of human knowledge. Such was the fate of Gilbert’s “effluvium” theory of electricity, of the fluid theories of Dufay and Franklin, and the æther-strain theory of recent years. “Each physical hypothesis,” says Prof. Fleming [associate and advisor to Marconi], “serves as a lamp to conduct us a certain stage in the journey. It illumines a limited portion of the path, throwing light before and behind for some distance ; but it has to be discarded and exchanged at intervals because it has become exhausted and because its work is done” [[xiv]].
The “radiation of a one charge accelerating” model makes no more sense than the sound of a single hand clapping. It may have illuminated our path for a while, but it has become exhausted and its work is done. John David Jackson (1925–2016), author of Classical Electrodynamics, the standard graduate level electromagnetism textbook used in American universities, observed:
It is evident that this manner of handling problems in electrodynamics can be of only approximate validity. The motion of charged particles in external force fields necessarily involves the emission of radiation whenever the charges are accelerated. The emitted radiation carries off energy, momentum, and angular momentum and so must influence the subsequent motion of the charged particles. Consequently the motion of the sources of radiation is determined, in part, by the manner of emission of the radiation. A correct treatment must include the reaction of the radiation on the motion of the sources.
Why is it that we have taken so long in our discussion of electrodynamics to face this fact? Why is it that many answers calculated in an apparently erroneous way agree so well with experiment? A partial answer to the first question lies in the second. There are many problems in electrodynamics which can be put with negligible error into one of the two categories described in the first paragraph. Hence it is worth while discussing them without the added and unnecessary complication of including reaction effects. The remaining answer to the first question is that a completely satisfactory treatment of the reactive effects of radiation does not exist [[xv]].
We will resume our discussion of electromagnetism and how it works in Chapter 6. First however, we must make a diversion into relativity and quantum mechanics to understand how else physics went wrong.
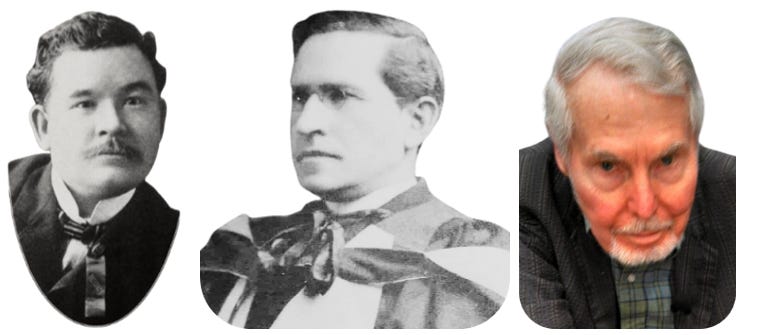
By the end of the nineteenth century, Platonism and the “atoms-and-void” model seemed to be in a general retreat in the realm of physics. Scientists generally accepted that there was some medium or physical process involved in the transmission of electromagnetic influences. Physicists sought to understand the nature of this process, in the Aristotelian spirit of looking to nature to infer, from her behavior, her form and structure on a more fundamental level.
In 1901, FitzGerald – like Maxwell – died of stomach problems. With Hertz gone, also, and with Heaviside and Lodge leaving the field to younger interlopers, the philosophic spirit of the Maxwellians would be supplanted by a younger generation, and a new and more virulent strain of Platonism would begin to infect physics.
Next time: 5.0 Relativity & Quantum Physics
Enjoy the article, but maybe not quite enough to subscribe?
Then click on the button below to buy me a coffee. Thanks!
Full Table of Contents [click here]
Chapter 4 Electromagnetism Comes of Age
4.7 Summary & Conclusions
Follow Online:
You may follow me online in other places as well:
Telegram: 𝔸𝕖𝕥𝕙𝕖𝕣𝕔𝕫𝕒𝕣'𝕤 𝔸𝕖𝕥𝕙𝕖𝕣𝕤𝕥𝕣𝕖𝕒𝕞
Gab: @aetherczar
Twitter: @aetherczar
Amazon: Hans G. Schantz
References
[[i]] Gilbert, William, de Magnete, P. Fleury Mottelay, translator, New York: John Wiley & Sons, 1893, p. 82. Originally published in Latin in 1600.
[[ii]] Gilbert, William, de Magnete, P. Fleury Mottelay, translator, New York: John Wiley & Sons, 1893, p. 176. Originally published in Latin in 1600.
[[iii]] “Drawing of an electrostatics experiment by English scientist Francis Hauksbee (or Hawksbee)(1660-1713) using an early electrostatic generator of his design, drawn by French clergyman and scientist Jean-Antoine Nollet, from his 1767 book Leçons de Physique. The electrostatic generator (right) consisted of a hollow ball of glass rotated by the man turning the crank. The friction of a man's hands on the ball created a charge of static electricity, which was conducted by the chain to the metal bar suspended by insulating silk cords. This experiment apparently demonstrated electrostatic induction. The woman (center) standing on a pad which insulated her from the ground, holding a metal plate in her right hand near another one hanging from the bar, had an electric charge induced in her body, which was communicated to the metal serving plate she is holding in her left hand. The man at right is performing some experiment with the charge; possibly demonstrating that fine powder sprayed on the plate is repelled into the air. Alternatively, the flask he is holding may be a Leyden jar which he is charging from the plate.
“The drawing illustrates how scientific research was conducted during the Enlightenment, as a cultured pastime of the genteel educated elite, performed in drawing rooms and salons, with women taking part.”
Downloaded July 28, 2013 from Agustin-Privat Deschanel (1889) Elementary Treatise on Natural Philosophy, 10th Ed., Part 3: Electricity and Magnetism, D. Appleton and Co., New York, p. 574, fig. 350 on Google Books. Credited in source to Abbé Nollet Leçons de Physique, 1767.
[[iv]] Ibid. See: https://commons.wikimedia.org/wiki/File:Hawksbees_Electrical_Machine_by_Jean-Antoine_Nollet.jpg
[[v]] Scientific Writings of Joseph Henry, vol. II, Washington: Smithsonian Institute, 1886, p. 312. See: https://books.google.com/books?id=w6cKAAAAIAAJ&newbks=1&newbks_redir=0&dq=%22sagacity%20and%20his%20power%20of%20scientific%20generalization%22&pg=PA312#v=onepage&q=%22sagacity%20and%20his%20power%20of%20scientific%20generalization%22&f=false
[[vi]] “Drawing of an electrostatics experiment by English scientist Francis Hauksbee (or Hawksbee)(1660-1713) using an early electrostatic generator of his design, drawn by French clergyman and scientist Jean-Antoine Nollet, from his 1767 book Leçons de Physique. The electrostatic generator (right) consisted of a hollow ball of glass rotated by the man turning the crank. The friction of a man's hands on the ball created a charge of static electricity, which was conducted by the chain to the metal bar suspended by insulating silk cords. This experiment apparently demonstrated electrostatic induction. The woman (center) standing on a pad which insulated her from the ground, holding a metal plate in her right hand near another one hanging from the bar, had an electric charge induced in her body, which was communicated to the metal serving plate she is holding in her left hand. The man at right is performing some experiment with the charge; possibly demonstrating that fine powder sprayed on the plate is repelled into the air. Alternatively, the flask he is holding may be a Leyden jar which he is charging from the plate.
“The drawing illustrates how scientific research was conducted during the Enlightenment, as a cultured pastime of the genteel educated elite, performed in drawing rooms and salons, with women taking part.”
Downloaded July 28, 2013 from Agustin-Privat Deschanel (1889) Elementary Treatise on Natural Philosophy, 10th Ed., Part 3: Electricity and Magnetism, D. Appleton and Co., New York, p. 574, fig. 350 on Google Books. Credited in source to Abbé Nollet Leçons de Physique, 1767.
[[vii]] See: https://commons.wikimedia.org/wiki/File:Pila_di_Volta_01.jpg
[[viii]] “Drawing of the first transformer, built by British scientist Michael Faraday in the 1830s. It consisted of an iron ring with two windings of insulated wire around it. Faraday attached one winding to a sensitive galvanometer. When he touched the other winding to a single cell battery, the winding created a changing magnetic field in the ring which induced a momentary current in the second winding due to electromagnetic induction, which was registered by the galvanometer.
“Although this device looks remarkably like a modern transformer, Faraday is not considered the inventor of the transformer. He only applied individual pulses of current to his device, not the alternating current that allows modern transformers to work continuously. More importantly the device had the same number of turns on both windings. Faraday did not discover the principle that makes transformers useful - that it can be used to transform the voltage up or down by using different numbers of turns in the secondary than in the primary winding.”
Downloaded 2009-08-06 from Frederick Bedell (1868-1958), 1896, The Principles of the Transformer, The MacMillan Co., New York, p. 288, fig. 162.
High resolution version from Friedrich Uppenborn (1859-1907), 1889, History of the Transformer, p 3, fig 2.
See: https://commons.wikimedia.org/wiki/File:Faradays_transformer.png
[[ix]] Lenard, Philipp, Great Men of Science: A History of Scientific Progress, translated from the German second edition by Dr. H. Stafford Hatfield, London: G. Bell and Sons, Ltd., 1958, p. 359.
[[x]] Lorentz, H.A. The Theory of Electrons, Leipzig: B.G. Teubner, 1909, p. 26.
[[xi]] Box, G. E. P. (1979), “Robustness in the strategy of scientific model building,” in Launer, R. L.; Wilkinson, G. N. (eds.), Robustness in Statistics, Academic Press, pp. 201–236, doi:10.1016/B978-0-12-438150-6.50018-2
[[xii]] Twitter/X post of August 31, 2024. See: https://x.com/skdh/status/1829813502669730149
[[xiii]] FitzGerald, George Francis, “Foundations of Physical Theory: Function of Models,” written no later than 1888, and collected in The scientific writings of the late George Francis FitzGerald, edited by Joseph Larmor, 1902.
[[xiv]] Irving, Edward, How to Know the Starry Heavens: An Invitation to the Study of Suns and Worlds, New York: Frederick A. Stokes Company, 1904, p. 190.
“We must bear in mind that scientific hypotheses as to the underlying causes of phenomena are subject to the law of evolution, and have their birth, maturity, and decay. Theory necessarily succeeds theory, and while no hypothesis can be looked upon as expressing the whole truth, neither is any likely to be destitute of all truth if it sufficiently reconciles a large number of observed facts.
“The notion that we can reach an absolutely exact and ultimate explanation of any group of physical effects is a fallacious idea. We must ever be content with the best attainable sufficient hypothesis that can at any time be framed to include the whole of the observations under our notice. Hence the question ‘What is electricity?’ no more admits of a complete and final answer to day than does the question, ‘What is life?’ Though this idea may seem discouraging, it does not follow that the trend of scientific thought is not in the right direction. We are not simply wandering round and round, chasing some illusive will-o’- the-wisp, in our pursuit after a comprehension of the structure of the Universe. Each physical hypothesis serves as a lamp to conduct us a certain stage on the journey. It illuminates a limited portion of the path, throwing a light before and behind for some distance, but it has to be discarded and exchanged at intervals because it has become exhausted and its work is done.” Professor J A Fleming
[[xv]] Jackson, John David, Classical Electrodynamics, 2nd ed., New York: John Wiley & Sons, 1975, pp. 780-781.
[[xvi]] The Creighton Chronicle: http://archive.org/stream/creightonchronic2n8crei#page/n1/mode/2up
See: https://infogalactic.com/w/images/c/cc/Photo_of_James_J._Walsh.jpg
[[xvii]] Anonymous, “Br Potamian O’Reilly: Celebrated Brother and scientist,” January 1, 2021. See: https://www.delasalle.org.uk/br-potamian-o-reilly-celebrated-brother-and-scientist
[[xviii]] “John David Jackson autographing a copy of his classical electrodynamics textbook.” This is a most unflattering photograph. While I am not a fan, I would have used a better photograph if one were available. See: https://en.wikipedia.org/wiki/John_David_Jackson_(physicist)#/media/File:Jackson_textbook_(cropped).jpg
This was one of the best serials on substack. Thank you for sharing your ideas so freely.
I immensely enjoy the contrasting symmetry and their profound consequences:
Electric energy - Magnetic energy = 0
Kinetic energy (space^2) - Potential energy (time^2) = 1